Research at WIPAC
At WIPAC, research focuses on particle astrophysics, which uses neutrinos, cosmic rays, and gamma rays to explore the extreme universe. Very powerful processes dominate the cosmos at energies above TeV, where particles are accelerated to energy levels much higher than those achievable with ground-based accelerators. This is what scientists call the nonthermal universe.
Astroparticle physicists use these naturally accelerated particles to look for an understanding of the mightiest environments in our universe. Although we know little about where and how nature generates the highest energy cosmic rays, photons, and neutrinos, primary suspects include supernovas black holes, gamma-ray bursts, and active galaxies. In this context, the search for dark matter is also of great interest.
WIPAC maintains and operates the IceCube Neutrino Observatory, located at the South Pole, under a cooperative agreement with the National Science Foundation. IceCube (link to project page) has been the main experiment that made WIPAC and the University of Wisconsin–Madison a vibrant place for astroparticle physics.
We also lead the effort to develop and construct the Askaryan Radio Array (ARA), also at the South Pole. We are collaborators of the dark matter detector DM-Ice (DM-Ice), the High-Altitude Water Cherenkov Observatory (HAWC) in Mexico, and the Cherenkov Telescope Array (CTA), to be built in Chile and the Canary Islands in Spain. Our researchers are also involved in the Dark Energy Survey (DES), the Fermi Gamma-ray Space Telescope, and the development of future instruments such as the CHIPS (CHerenkov Detectors in Mine Pits) project, which deployed its first prototype detector in a former iron mine in northern Minnesota in August 2015.
Neutrino Astronomy
After decades of expanding our knowledge by exploring further frequencies of electromagnetic radiation, the study of the extreme universe has produced new and hard-to-address challenges. Having no electric charge and essentially no mass, a neutrino is much like a photon; it differs in one important attribute—its interactions with matter are very feeble. Neutrinos go through walls; light does not.
In fact, neutrinos can penetrate matter through vast distances. This allows us to explore the inside regions of the most violent cosmic environments, such as the inner neighborhoods of black holes, which are opaque to electromagnetic radiation. However, neutrinos are notoriously difficult to detect.
In 1960, Kenneth Greisen, Moisey Markov and Fred Reines proposed the first detectors to use neutrinos for astronomy. Since then, neutrino detectors have “seen” the Sun, also proving that neutrinos have a tiny mass, and they even detected a supernova in the Large Magellanic Cloud in 1987.
Because of the weak interaction with matter, neutrino detectors are huge, and their sizes increase in order to explore the universe at even higher energies. To observe “Greisen-Zatsepin-Kuzmin” (GZK) neutrinos, which are produced in the interactions of ultra-high-energy cosmic ray protons with microwave photons left over from the Big Bang, we need to build kilometer-scale detectors.
In the same way that only nature can accelerate particles to ultrahigh energies, only nature can provide the medium to detect cosmic neutrinos. After the first attempts with air and water, Francis Halzen and John Learned proposed using ice in the late 1980s. The South Pole now hosts the only cubic-kilometer neutrino detector constructed to date, IceCube, which transformed a cubic kilometer of the Antarctic ice shelf into such a neutrino detector.
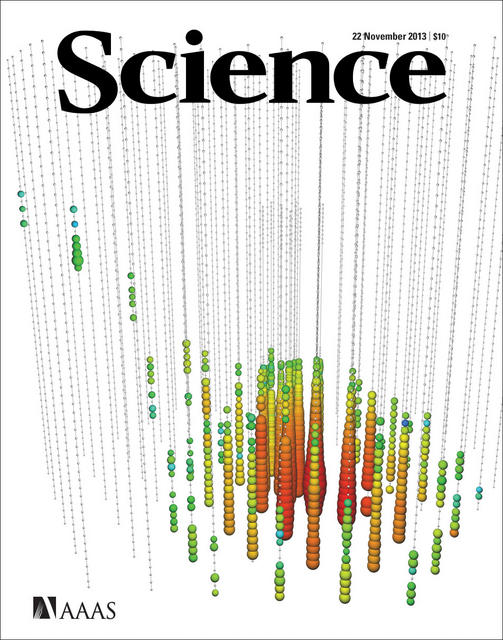
IceCube was based on previous efforts of a growing neutrino astronomy community. The Deep Underwater Muon and Neutrino Detector (DUMAND) in the sea off the main island of Hawaii pioneered many of the detector technologies in use today. It also inspired the deployment of a neutrino detector in Lake Baikal as well as efforts in the Mediterranean. An initiative launched at UW–Madison in the late 1980s, which led to the creation of WIPAC, continued the quest for the first telescope on the scale envisaged by the DUMAND collaboration but using a large volume of deep Antarctic ice instead.
The Antarctic Muon and Neutrino Detector Array (AMANDA), in operation from 2000 to 2009, represented the proof of concept for the first kilometer-scale neutrino observatory, IceCube, completed in 2010. The IceCube detection of a flux of neutrinos of PeV energy reaching us from the cosmos finally launched high-energy neutrino astronomy.
WIPAC researchers are also pioneering the observation of ultra-high-energy neutrinos using the emission of radio waves produced by neutrino interactions with Antarctic ice. The Askaryan Radio Array (ARA), currently under construction at the South Pole, is a scalable and cost-effective detector for pursuing the search for GZK neutrinos.
Cosmic-Ray Astronomy and Cosmic-Ray Physics
Cosmic-ray astronomy started with the goal of discovering the sources of the universe’s highest energy particles. High-energy cosmic rays have been known to exist since the early 1900s, but their origin remains one of the oldest science conundrums.
Using cosmic rays to explore the universe opened up astronomy to new techniques. Cosmic rays are the only charged particles used in astronomy, whereas electromagnetic radiation, neutrinos, or even gravitational waves are neutral messengers.
Cosmic-ray trajectories are scrambled by magnetic fields as they travel throughout the Milky Way, and these cosmic particles end up bombarding the Earth from almost every direction. However, for energies above several EeV, the deviation for most cosmic rays is expected to be only a few degrees, and strong nearby sources could be observed as a high-density cosmic-ray cluster in the sky. The detection of these sources should be even easier at energies above 50 EeV, since cosmic rays from sources beyond 10 Mpc are expected to be absorbed by the cosmic microwave background, as is the case for gamma rays, which are also seen by many cosmic-ray detectors. Our galaxy is approximately 30 kpc long and about 0.3 kpc thick.
Astrophysicists have also observed small anisotropies in the arrival direction of cosmic rays at energies below EeV, which could be related to the diffusion of cosmic rays from nearby sources or the magnetic fields of the heliosphere.
Finally, the energy spectrum and chemical composition of cosmic rays are also powerful tools for studying the nature of astrophysical accelerators, the processes that power these mighty cosmic engines.
WIPAC researchers are involved in searches for cosmic-ray sources, studies of the cosmic-ray anisotropy, and the precise measurement of the cosmic-ray flux and its chemical composition using data from IceCube and HAWC. Results include the first measurement of the cosmic-ray anisotropy in the Southern Hemisphere with IceCube, expanding the search for anisotropies up to PeV energies.
Gamma-Ray Astronomy
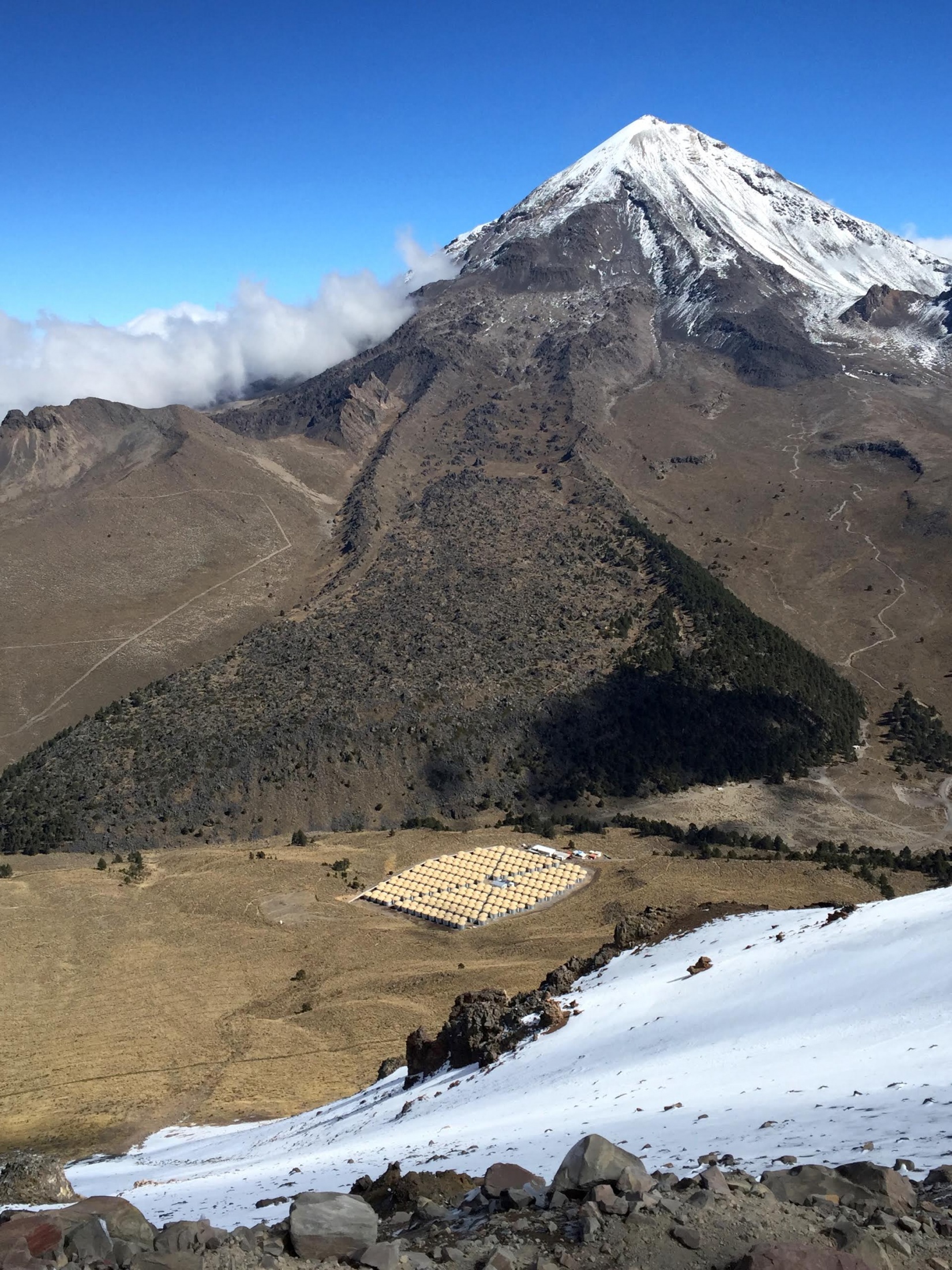
Gamma rays give a complementary view of the extreme universe to those obtained with neutrinos and cosmic rays. And although they might not be the best messengers for exploring the universe at the highest energies, they are outstanding messengers for the still almost unexplored TeV universe.
Gamma-ray absorption limits the horizon of the universe that can be explored. At 1 TeV, the visible universe is limited to 500 Mpc around the detector location, mainly due to the interactions with starlight. Above several tens of TeV, the limiting factor is the interactions with extragalactic photon fields, such as the infrared and microwave backgrounds, limiting the visible universe to a few kpc.
HAWC is designed, as CTA will be in the future, to play a major role in gamma-ray astronomy. IceTop, the surface array of the IceCube Neutrino Observatory, is the most sensitive detector of PeV gamma rays in the Southern Hemisphere. Highlights of our research on gamma rays include a study of extragalactic TeV gamma-ray sources in HAWC, in particular the real-time search for flaring activity in active galactic nuclei (AGN). In IceCube, we are currently developing a measurement of the gamma-ray flux, which if found would provide support for a galactic component of the observed astrophysical neutrino flux.
Dark Matter Searches
Dark matter is estimated to make up nearly one quarter of all the matter and energy of the universe. Scientists have observed the effects of dark matter in the dynamics of galaxies, which cannot be explained with the visible matter in these environments. However, there is no definitive evidence of the existence of dark matter or of what kind of particles constitute it. WIPAC is involved in both direct and indirect dark matter searches. In direct searches, we are looking for the signature of a dark matter particle interaction in our detector, while for indirect searches we look for energetic particles that would be created if dark matter annihilates or decays in cosmic environments.
WIPAC played a main role in the design and construction of the DM-Ice detector, a dark matter detector in Antarctica, and currently contributes to its operations and data analysis. DM-Ice aims to carry out a definitive test of the only claim to have seen a dark matter signal to date. The DAMA experiment, at the Gran Sasso National Laboratory in Italy, reported the observation of an annual modulation in the number of observed dark matter induced interactions in the DAMA detector. For DM-Ice, located at the South Pole, the phase of environmental backgrounds is reversed compared to DAMA’s in the Northern Hemisphere, while the dark matter signature is the same in both hemispheres. The current DM-Ice17 is a prototype for a full 250 kg-scale crystal array. WIPAC researchers are currently studying detectors with lower backgrounds and optimizing the design of the future DM-Ice250.
WIPAC researchers in DES are finding new ultrafaint galaxies orbiting the Milky Way. These galaxies are excellent targets for indirect dark matter searches using gamma-ray and neutrino telescopes because of their proximity, large dark matter content, and low conventional astrophysical backgrounds. The recent discovery of more than 20 ultrafaint galaxy candidates will enhance the sensitivity of this dark matter search strategy.
Our CTA team is developing a technique to measure the positron flux at Earth using the “moon shadow” as a way to distinguish electrons from oppositely charged positrons. An excess of high-energy antimatter particles, such as positrons, is one predicted signature of dark matter annihilation or decay.
Multimessenger Astronomy and Theoretical Astrophysics
WIPAC researchers, together with other collaborators, are constantly interpreting new data from neutrino, cosmic-ray, and gamma-ray detectors and extracting all of the physics potential from combined data. In particular, these multimessenger studies can provide some insight into the origin of the astrophysical neutrino flux recently discovered by IceCube. For instance, they show the absence of a strong correlation between neutrino events and Galactic TeV gamma-ray emission and that the upper limit on Galactic PeV gamma-ray emission constrains the Galactic neutrino origin. Researchers at WIPAC are also probing the extragalactic GeV-TeV gamma-ray background for multimessenger emission of distant neutrino sources, to potentially derive general theoretical constraints on neutrino production mechanisms in the extragalactic source candidates. These studies have also shown that starburst galaxies—one of the usual suspects for neutrino emission—are unlikely to be the main source of the IceCube observation. In this manner, the small theoretical team, led by Prof. Francis Halzen, plays a strong contributing role in the growing international astrophysics phenomenology community.
One of the highlights of this effort is the biennial IceCube Particle Astrophysics (IPA) symposium, which brings together theoreticians and experts from other experiments to discuss the present state and future of neutrino astronomy and related topics of multiwavelength cosmic messengers, neutrino properties, and cosmological and dark matter aspects of particle astrophysics.